Fitness
Spinal infections? mNGS combined with microculture | IDR
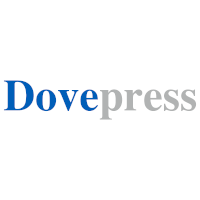
Background
Spinal infections, which can involve bacterial, viral, or fungal attacks on spinal structures and surrounding tissues, may result in inflammation and damage to bones, intervertebral discs, or soft tissues.1,2 The overall incidence of spinal infections is approximately 2.2/100,000 per year.3 In recent years, there has been a consistent rise in incidence due to factors such as an aging population, the widespread performance of spinal surgeries, and an increase in immunodeficiency diseases like HIV.4,5 The infection can spread through the bloodstream or invade the spine directly. Symptoms in patients vary depending on the severity and location of the infection and commonly include back pain, fever, localised redness and swelling, restriction of movement and spinal deformity. If the infection spreads to the spinal cord or nerve roots, it may also cause limb weakness, sensory abnormalities, or urinary difficulties.1,3,6 Due to the low specificity of signs and symptoms, diagnosis may be delayed or inaccurate, increasing the risk of misdiagnosis or missed diagnosis3,6,7, which may exacerbate the patient’s prognosis.8 Thus, this makes early and accurate diagnosis a clinical challenge. Metagenomic Next-Generation Sequencing (mNGS), an emerging technology, has demonstrated its potential in the identification of pathogens in cases of infection, and has been reported to allow for unbiased sampling, broad and rapid identification of known pathogens, and even the discovery of new microorganisms.9,10 It has been shown that mNGS has applications in the diagnosis and treatment of infectious diseases, including spinal infections.11 mNGS helps spine surgeons at the diagnostic stage, helping them to identify appropriate treatment options as early as possible.12 However, research on pathogen detection using mNGS in spinal infections remains limited, and the therapeutic value needs further clarification. Therefore, our study aimed to assess the capability of metagenomic next-generation sequencing (mNGS) to identify the etiology of spinal infections, explore its impact on treatment planning when combined with microbiological culture and pathology, and investigate post-treatment changes in blood test markers and clinical efficacy.
Methods and Materials
Methods of Study
Inclusion criteria: (1) Patients preliminarily diagnosed with spinal infection based on clinical signs, laboratory findings, and imaging studies.7,13 (2) Samples obtained using C-arm X-ray guided puncture or surgery. (3) At least two different diagnostic methods used for analyzing tissue samples. Exclusion criteria: (1) Only one diagnostic method used for sample testing. (2) Samples evidently contaminated during submission. (3) Incomplete clinical data or patient lost to follow-up. (4) Follow-up period less than three months. According to the above inclusion and exclusion criteria, a total of 46 patients were included in this study (Figure 1). The study consisted of 23 males and 23 females, with the median age being 63 years old (interquartile range: 12–86 years). This study was approved by the Ethics Committee of the Second Affiliated Hospital of Guangxi Medical University.
Figure 1 Flow chart. |
Detailed clinical data were collected from the patients, including WBC, neutrophil ratio, CRP, ESR, PCT, initial Visual Analogue Scale (VAS) scores, and imaging findings. Lesion tissue, peri-lesional soft tissue, or pus samples were obtained via C-arm X-ray assisted puncture or open surgery, then sealed in sterile culture tubes and sent for immediate postoperative examination for mNGS, routine microbiological culture, or pathological analysis, respectively. Customized antibacterial treatment plans were devised for infected patients based on their clinical symptoms, imaging results, mNGS, bacterial culture, or histopathological findings. A follow-up assessment was conducted on day 7 postoperatively. The sensitivity and specificity of mNGS for detecting spinal infection pathogens were evaluated, as well as its impact on the treatment process and prognosis, according to the final clinical outcomes. Demographic and clinical information was sourced from the electronic medical records of the Second Affiliated Hospital of Guangxi Medical University. mNGS testing, routine microbiological cultures and pathological analyses were performed in-house by our laboratory.
mNGS Testing and Analysis
The samples were stored at low temperature, and the DNA was extracted and purified by magnetic bead method according to the Microbial DNA Extraction Kit (Yugo Zhizhi Technology Co., Ltd., China), and then the macro-genomic library was constructed according to the Library Construction Kit (Yugo Zhizhi Technology Co., Ltd., China) (library size: 330–350bp), and quantified by using the Nucleic Acid Quantification System Qubit 4.0 (Thermo Fisher Scientific, USA). The libraries with different sequence tags were mixed in equal quantities, and high-throughput sequencing was completed using the Illumina NextSeq CN500 (Illumina Inc., USA) sequencing platform. The data were basically filtered by Fast QC software, including removing sequences containing sequencing junctions, sequences containing more than 10% of data, and sequences containing more than 50% of low-quality bases (Q value ≤ 10), and then the filtered data were used to perform BWA comparison with the human genome reference sequences, removing human-related sequences. Microbial sequences were then compared and annotated against an optimized pathogen database provided by Yugo Zhizhi Technology Co., Ltd., completing the result analysis. The laboratory procedure for the sample is shown in Figure 2.
![]() |
Figure 2 Flow chart of mNGS. |
Statistical Analysis
The final clinical diagnosis was used as the gold standard. The McNemar test was employed to assess significant differences in sensitivity, specificity, PPV, and NPV. The detailed calculations are provided in Supplementary (Supplement 1). Data adhering to a normal distribution were presented as mean ± standard deviation and compared using the t-test. Conversely, data not following a normal distribution were described by the median and interquartile range and assessed with the Mann–Whitney U-test for comparison. A p-value of
Results
General Clinical Data Comparison Were Shown inTable 1
A total of 46 patients suspected to have spinal infection in our hospital between January 1, 2022 and December 30, 2023 were included. They included 23 males and 23 females, aged between 12 and 86 years, with a mean of (61.67±14.48) years. Pus or tissue specimens were obtained by X-ray C-arm underguided puncture in 26 cases, purulent tissue or pus specimens were obtained by open surgery in 18 cases, and specimens were obtained by spinal endoscopy in two other cases. By evaluating the history, clinical symptoms, physical examination findings, laboratory test data, imaging data, and surgical findings, 32 cases were diagnosed as spinal infections, while 11 were diagnosed as noninfectious, 1 as a tumor, and 2 could not be diagnosed. Fourteen of the included cases had been treated with antibiotics within 30 days prior to admission. TB-33 eventually died. At the final follow-up, all other patients demonstrated favorable recovery outcomes.14,15
![]() |
Table 1 Baseline Characteristics of Participants by Infection Status |
Comparison of mNGS, Microbial Culture, and PCT
A total of 52 samples were analyzed, including 10 pus and secretion samples and 42 tissue samples. Of the 49 samples submitted for mNGS, 30 tested positive and 19 negative, yielding a positivity rate of 61.2% (30/49) (Table 2). Seven samples were only tested with mNGS and not with conventional microbial culture due to limited tissue and pus availability. The positivity rate of conventional microbial culture was 30.8% (12/39). The positivity rate of mNGS was notably greater compared to the conventional microbial culture and PCT, with a statistically significant difference. In clinically diagnosed specimens, the positivity rates for mNGS of tissue and pus samples were 79.3% and 80% respectively, showing no statistically significant difference (p > 0.99). The positivity rates for conventional microbial culture of tissue and pus samples were 25% and 62.5% respectively (p = 0.088), which is not a statistically significant difference. These Results indicate that the type of sample did not affect the positivity rates of mNGS and conventional microbial culture. Detailed test results are contained in Supplement 2.
![]() |
Table 2 Comparison of mNGS, Microbial Culture, and PCT Positivity Rates |
Comparison of Diagnostic Efficacy
Comparative analysis showed mNGS with a sensitivity of 79.41% and specificity of 80%, outperforming the conventional microbial culture’s sensitivity of 25%, albeit with a specificity of 100% (Table 3). This indicates mNGS’s superior sensitivity. Out of 32 patients diagnosed with spinal infection, mNGS identified 27 positive cases (accounting for 62.8%), while conventional culture detected only 8 positives (18.6%). Complete concordance was observed in 4 patients. Additionally, 4 patients showed partial concordance, with at least one pathogen identified by mNGS also confirmed by culture, with no discrepancies between the two methods’ results (Figure 3A). Furthermore, the chart depicts the time required to acquire results from mNGS, culture, and pathology (Figure 3B).
![]() |
Table 3 Sensitivity and Specificity of mNGS Compared with Cultures |
![]() |
Figure 3 (A) The concordance of mNGS and microbial culture in detecting pathogenic microorganisms. (B)Time cost of mNGS, culture and pathology. |
Detection Outcomes
A total of 49 mNGS tests were performed in 46 patients (in 2 of these patients, tissue specimens obtained by puncture on admission were negative for mNGS testing, but tissue specimens taken during open surgery turned out to be positive again). Surprisingly, among these microorganisms, Mycobacterium tuberculosis was detected in the highest number, 6 times, accounting for 20% (6/30) of the total number of positive detections. Among purulent bacteria, Gram-positive and Gram-negative bacteria were predominantly Staphylococcus aureus (detected 5 times) and Escherichia coli (also detected 5 times). Additionally, Brucella ovis were identified 4 times. Other less common bacteria, fungi, and viruses such as Aspergillus fumigatus, Malassezia furfur, Hepatitis E virus, and Human herpesvirus 5 were also detected (detailed data available in Figure 4A and B). In routine microbiological assays, the highest detection rate was for the Gram-positive bacterium Staphylococcus aureus.
![]() |
Figure 4 Pathogenic microorganisms detected by mNGS. (A) Pathogenic microorganisms detected in all samples(B)Background microbial distribution. |
Out of 7 patients diagnosed with Mycobacterium tuberculosis infection, all tested positive via T-spot. Six underwent histopathological examination, with all six yielding positive results. mNGS results were positive in six cases and negative in one, with no positive outcomes from culture. A patient with Malassezia furfur infection initially tested negative in routine microbial culture, but after a positive mNGS result, subsequent samples confirmed positive with targeted microbial culture.
Follow-Up Status
In this study, of the patients with confirmed spinal infections, 26 patients underwent surgical treatment. Based on mNGS results, microbial cultures, and pathological analysis, we tailored antimicrobial treatment plans for the patients (Figure 5). Specific imaging and pathology of typical cases can be found in Supplement (Supplement 3 and Figures S1–S6). Patients infected with Mycobacterium tuberculosis were treated with “quadruple therapy” (isoniazid, rifampicin, pyrazinamide, ethambutol) for at least 12 months.16 Patients infected with Staphylococcus aureus were treated with cefotaxime sodium, vancomycin, linezolid or moxifloxacin. Patients infected with Brucella suis received doxycycline, streptomycin, or rifampin. One additional patient with Aspergillus fumigatus infection was treated with voriconazole. Patients with Streptococcus suis infection were treated with ceftriaxone sodium, levofloxacin tablets linezolid tablets, while patients with Serratia mucinosa and human cytomegalovirus infection were treated with meropenem, compound sulfamethoxazole tablets. The duration of antibiotic therapy was maintained for at least 6 weeks in all patients. Patients infected were followed up at seven days of drug or surgical treatment. As shown in figure (Table 4), there was a decrease in leukocytes, sedimentation and C-reactive protein values in patients with spinal infection after seven days of treatment. The decrease in neutrophil ratio was statistically significant. All patients were followed up after treatment. Follow-up period was (8.5 ± 4.1) months. During this period, three patients experienced recurrence of infection but subsequently improved; one patient died and the others were in good condition. The VAS scores, ODI and JOA scores of the patients at the time of follow-up are shown in the figure (Table 5). These scores improved significantly after treatment and the changes were statistically significant.
![]() |
Table 4 Comparison of Patients with Confirmed Infection Pre-Treatment and After Treatment |
![]() |
Table 5 Comparison of All Patients Pre-Treatment and After Treatment |
![]() |
Figure 5 Application of mNGS in Clinical Diagnosis and Therapeutic Decision-Making. In 32 confirmed infection cases, treatment plans were formulated or adjusted for 27 patients based on mNGS results. |
Discussion
Conclusive evidence of spinal infection is predicated upon the successful isolation of pathogens via conventional microbiological culturing techniques. Nonetheless, the efficacy of these cultures is compromised by their low yield, the extended duration required for pathogen identification, and the possible influence of preceding antibiotic treatments,17,18 Even with the methodological advancements proposed by Peel17 and Schafer19, such as prolongation of culture duration and refinement of detection techniques, certain pathogens continue to evade identification. Pathological examination is regarded as the “gold standard” for the confirmation of spinal infection diagnoses but is insufficient for detecting low-virulence microbial infections and cannot provide specific information of pathogenic bacteria.20 Specific PCR assays have been reported to have high sensitivity but are unable to cover rare and emerging pathogens.10,21 This poses a challenge to spine surgeons: how to identify pathogens early and quickly, enabling rapid and precise treatment. Metagenomic sequencing (mNGS), as an emerging non-culture-based technology with high sensitivity and specificity, fast detection and less affected by pre-sampling antibiotics,22,23 has shown higher sensitivity than traditional culture-based methods in the detection of pathogens in bloodstream infections, lung infections, and other diseases in terms of confirming infections and their causative organisms.24–26In this paper, the positive detection rate of mNGS (61.2%) was significantly higher than that of routine microbiological culture (30.8%) and calcitoninogen assay (28%), in line with the findings of a previous study.27 Notably, 17 culture-negative patients presented positive results by mNGS. mNGS detected Mycobacterium tuberculosis, Brucella suis and Aspergillus fumigatus, which confirms that mNGS surpasses conventional microbiological cultures in terms of detection efficacy. In terms of detection time, conventional microbiological culture took (3.09±1.16) days and pathology took (2.68±1.85) days, while mNGS took (1.54±0.75) days, showing its obvious advantage in time efficiency. Specific spinal infections include tuberculosis, Brucella, fungal, and viral infections. However, in this study, only one case of infection with Malassezia sympodialis was culture-positive, indicating the low sensitivity of conventional culture methods in diagnosing specific spinal infections. Fortunately, mNGS detection rates exceed 90%, demonstrating its significant potential as an effective tool for diagnosing specific spinal infections. Additionally, the research highlights Staphylococcus aureus as the most common pathogen in pyogenic spinal infections, with other opportunistic pathogens such as Stenotrophomonas maltophilia and Streptococcus intermedius also detected, previously reported in spinal infections.28,29 It is noteworthy that in this study, multiple patient samples were found to contain Veillonella parvula, which is considered to be associated with spondylodiscitis,30 Metagenomic Next-Generation Sequencing (mNGS) identified it as a Background microbial infection, the results underscore the unique advantage of mNGS in identifying rare pathogens. mNGS can be used as an aid to provide additional information on the pathogen to help doctors make more comprehensive diagnosis and treatment decisions. In the early stages of disease diagnosis, mNGS offers rapid and accurate information, assisting clinicians in devising early, targeted antibiotic treatments to prevent antibiotic misuse.31,32 In this study, antibiotic regimens were adjusted for 27 patients diagnosed with infections based on mNGS results. Furthermore, studies indicate that combining mNGS with microbiological culture and pathological examination can more effectively bolster clinicians’ confidence when making decisions, as compared to relying solely on mNGS results. Initially suspected of tuberculosis, patient T-30 tested negative in both puncture culture and mNGS, while pathological examination revealed signs of metastatic prostate cancer. Consequently, relevant tumor markers were further investigated, leading to the final diagnosis of the tumor. T-31 postoperative culture results were negative, pathological examination showed the presence of inflammatory cell infiltration in the bone marrow cavity, and mNGS test suggested Brucella infection. Based on these results, we ultimately developed a targeted antibiotic regimen for the patient with a combination of doxycycline and rifampicin. In a case of Malassezia furfur infection, initial microbiological culture was negative, mNGS testing positive, and histopathology showed extensive plasmacytic and lymphocytic infiltration in bone tissue. Following these outcomes, cultures were redone under specific conditions, ultimately confirming a positive result. This study also examined the effectiveness of treatment regimens combining mNGS, microbiological culture and pathological findings in the management of spinal infections and their impact on prognosis. For these reasons, all patients in the study were followed up, revealing significant improvement in prognostic indicators.
The application of mNGS currently has certain limitations. Firstly, the method and location of sample collection during the preparation stage may affect the outcomes. T18 and T46 had negative mNGS results on the first puncture for tissue samples and positive results after the second open surgery for tissue samples. This suggests that sampling method and site may have a potential impact on mNGS outcomes, a topic not yet thoroughly investigated in the literature. This study attempted to explore the impact of pus and tissue sample types on the positive rate of mNGS, but the findings were not statistically significant, aligning with previous research.33 Additionally, the high sensitivity of mNGS may also lead to a higher false-positive rate, which might contribute to the lower specificity of mNGS compared to traditional microbial cultures observed in this study. Finally, there is a time lapse from sample collection to result analysis, rendering mNGS potentially unsuitable in certain urgent scenarios. Despite current limitations in mNGS application, ongoing technological advancements and improvements are expected to progressively resolve these issues.
Conclusion
mNGS demonstrates enhanced sensitivity in detecting pathogens in spinal infections, especially rare and critical ones, compared to traditional microbial culture. Based on these findings, we propose that mNGS can serve as a valuable adjunct in enhancing the diagnostic process of spinal infections. Although mNGS cannot fully replace microbial culture, its integration with conventional methods offers a comprehensive approach to diagnosing and treating spinal infections. This combined strategy can lead to more personalized and effective therapeutic regimens, ultimately improving patient outcomes. This study’s single-center, retrospective design with a limited sample size may introduce bias. Future research across multiple centers with larger sample sizes is anticipated to corroborate our findings, and an extended follow-up period is desired to more comprehensively assess the impact of mNGS-guided treatment on patient recovery and clinical outcomes.
Data Sharing Statement
The datasets used and/or analyzed during the present study are available from the corresponding author on reasonable request.
Ethical Approval and Consent to Participate
We confirm that all patients and legal guardian of the patient who died in this research obtained informed consent. We confirm that all three patients whose case details are described in the Supplementary File have provided written informed consent for the publication of their case details and images. We confirm that a parent or legal guardian of patients under 18 years of age provided has provided informed consent. The research was approved by the Ethics Committee of the Second Affiliated Hospital of Guangxi Medical University in accordance with the Helsinki Declaration of the World Medical Association and the ethical principles formulated by the Chinese GPC.
Consent for Publication
Informed consent was obtained from all individual participants included in this study.
Acknowledgments
We are grateful to Dr. Yuanming Chen (The Second Affiliated Hospital of Guangxi Medical University) for his kindly assistance in all stages of the present study.
This paper has been uploaded to Research Square as a preprint: https://www.researchsquare.com/article/rs-3950629/v1.
Jiayi Chen and Yonghong Liu should be regarded as co-first authors.
Disclosure
The authors declare that they have no competing interests.
References
1. Babic M, Simpfendorfer CS. Infections of the Spine. Infect Dis Clin North Am. 2017;31(2):279–297. doi:10.1016/j.idc.2017.01.003
2. Duarte RM, Vaccaro AR. Spinal infection: state of the art and management algorithm. Eur Spine J. 2013;22(12):2787–2799. doi:10.1007/s00586-013-2850-1
3. Tsantes A, Papadopoulos D, Vrioni G, et al. Spinal infections: an update. Microorganisms. 2020;8(4):476. doi:10.3390/microorganisms8040476
4. Chakaya J, Khan M, Ntoumi F, et al. Global tuberculosis report 2020 – reflections on the global TB burden, treatment and prevention efforts. Inter J Infect Dis. 2021;113:S7–S12.
5. Chuo C-Y, Y-C F, Y-M L, et al. Spinal Infection in Intravenous Drug Abusers. J Spinal Disord Tech. 2007;20(4):324–328. doi:10.1097/BSD.0b013e31802c144a
6. Lener S, Hartmann S, Barbagallo GMV, Certo F, Thomé C, Tschugg A. Management of spinal infection: a review of the literature. Acta Neurochirurgica. 2018;160(3):487–496. doi:10.1007/s00701-018-3467-2
7. Berbari EF, Kanj SS, Kowalski TJ, et al. 2015 Infectious Diseases Society of America (IDSA) clinical practice guidelines for the diagnosis and treatment of native vertebral osteomyelitis in adultsa. Clinl Infect Dis. 2015;61(6):e26–e46. doi:10.1093/cid/civ482
8. Schwab JH, Shah AA. Spinal epidural abscess: diagnosis, management, and outcomes. J Am Acad Orthop Surg. 2020;28(21):e929–e938. doi:10.5435/JAAOS-D-19-00685
9. Gu W, Miller S, Chiu CY. Clinical metagenomic next-generation sequencing for pathogen detection. Ann Rev Pathol. 2019;14(1):319–338. doi:10.1146/annurev-pathmechdis-012418-012751
10. Miller S, Chiu C. The role of metagenomics and next-generation sequencing in infectious disease diagnosis. Clin. Chem. 2022;68(1):115–124. doi:10.1093/clinchem/hvab173
11. Simner PJ, Miller S, Carroll KC. Understanding the promises and hurdles of metagenomic next-generation sequencing as a diagnostic tool for infectious diseases. Clinl Infect Dis. 2018;66(5):778–788. doi:10.1093/cid/cix881
12. Zhang G, Zhang H, Hu X, et al. Clinical application value of metagenomic next-generation sequencing in the diagnosis of spinal infections and its impact on clinical outcomes. Front Cell Infect Microbiol. 2023;2023:13.
13. Ortiz AO, Levitt A, Shah LM, et al. ACR appropriateness criteria® suspected spine infection. J Am College Radiol. 2021;18(11):S488–S501. doi:10.1016/j.jacr.2021.09.001
14. Yagdiran A, Sircar K, Jung N, Eysel P, Bredow J, Beyer F. Determining threshold values for success after surgical treatment of lumbar spondylodiscitis using quality of life scores. Acta Orthopaed et Traumatolog Turci. 2023;57(3):99–103. doi:10.5152/j.aott.2023.22137
15. Yoon SH, Chung SK, Kim K-J, Kim H-J, Jin YJ, Kim HB. Pyogenic vertebral osteomyelitis: identification of microorganism and laboratory markers used to predict clinical outcome. Eur Spine J. 2009;19(4):575–582. doi:10.1007/s00586-009-1216-1
16. Jain AK, Rajasekaran S, Jaggi KR, Myneedu VP. Tuberculosis of the Spine. J Bone Joint Surg Am. 2020;102(7):617–628. doi:10.2106/JBJS.19.00001
17. Peel TN, Dylla BL, Hughes JG, et al. Improved diagnosis of prosthetic joint infection by culturing periprosthetic tissue specimens in blood culture bottles. mBio. 2016;7(1). doi:10.1128/mBio.01776-15
18. Cornett CA, Vincent SA, Crow J, Hewlett A. Bacterial spine infections in adults. J Am Acad Orthop Surg. 2016;24(1):11–18. doi:10.5435/JAAOS-D-13-00102
19. Schäfer P, Fink B, Sandow D, Margull A, Berger I, Frommelt L. Prolonged bacterial culture to identify late periprosthetic joint infection: a promising strategy. Clinl Infect Dis. 2008;47(11):1403–1409. doi:10.1086/592973
20. Bürger J, Palmowski Y, Strube P, Perka C, Putzier M, Pumberger M. Low sensitivity of histopathological examination of peri-implant tissue samples in diagnosing postoperative spinal implant infection. Bone Joint J. 2020;102-B(7):899–903. doi:10.1302/0301-620X.102B7.BJJ-2019-1725.R2
21. Afshari A, Schrenzel J, Ieven M, Harbarth S. Bench-to-bedside review: rapid molecular diagnostics for bloodstream infection – a new frontier? Critical Care. 2012;16(3):222. doi:10.1186/cc11202
22. Miao Q, Ma Y, Wang Q, et al. Microbiological diagnostic performance of metagenomic next-generation sequencing when applied to clinical practice. Clinl Infect Dis. 2018;67(suppl_2):S231–S240.
23. Shangguan L, Xue L, Shang J, Wang H. The application value of metagenomic next-generation sequencing in community-acquired purulent meningitis after antibiotic intervention. BMC Infect Dis. 2023;23(1). doi:10.1186/s12879-023-08672-4
24. Sun L, Zhang S, Yang Z, et al. Clinical application and influencing factor analysis of metagenomic next-generation sequencing (mNGS) in ICU patients with sepsis. Front Cell Infect Microbiol. 2022;2022:12.
25. Jin X, Li J, Shao M, et al. Improving suspected pulmonary infection diagnosis by bronchoalveolar lavage fluid metagenomic next-generation sequencing: a multicenter retrospective study. Microbiol Spect. 2022;10(4). doi:10.1128/spectrum.02473-21
26. Chen H, Zhang Y, Zheng J, et al. Application of mNGS in the etiological diagnosis of thoracic and abdominal infection in patients with end-stage liver disease. Front Cell Infect Microbiol. 2022;11:1.
27. Zhang H-C, J-W A, Cui P, et al. Incremental value of metagenomic next generation sequencing for the diagnosis of suspected focal infection in adults. J Infect. 2019;79(5):419–425. doi:10.1016/j.jinf.2019.08.012
28. Pralea A, Walek KW, Auld D, Mermel LA. Differences in microorganisms causing infection after cranial and spinal surgeries. J Neurosurg. 2023;2023:1–8.
29. Olmos MA, González AS, Clemente JD, Tomé CV. Infected vertebroplasty due to uncommon bacteria solved surgically: a rare and threatening life complication of a common procedure. Spine. 2006;31(20):E770–E773. doi:10.1097/01.brs.0000240202.91336.99
30. Kierzkowska M, Pedzisz P, Babiak I, et al. Orthopedic infections caused by obligatory anaerobic Gram-negative rods: report of two cases. Med Microbiol Immunol. 2017;206(5):363–366. doi:10.1007/s00430-017-0513-2
31. Zhao Y-C, Ding Y-Z, Zhao X, et al. Role and clinical application of metagenomic next-generation sequencing in immunocompromised patients with acute respiratory failure during veno-venous extracorporeal membrane oxygenation. Front Cell Infect Microbiol. 2022;2022:12.
32. Sun T, Wu X, Cai Y, et al. Metagenomic next-generation sequencing for pathogenic diagnosis and antibiotic management of severe community-acquired pneumonia in immunocompromised adults. Front Cell Infect Microbiol. 2021;2021:11.
33. Zhang Y, Chen J, Yi X, et al. Evaluation of the metagenomic next-generation sequencing performance in pathogenic detection in patients with spinal infection. Front Cell Infect Microbiol. 2022;2022:12.